Quantum Mechanical Electron Oscillations Observed in Molecules
- Research
- Top News

Junior professor Wolfram Helml from the Department of Physics at TU Dortmund University, together with an international research team, has made new observations in the field of photochemistry of molecular quantum systems. Using a “stopwatch” for ultrafast electron processes in atoms, they have succeeded in observing and controlling coherent superpositions of nuclear excited states in molecules. Scientists from research institutes in the USA, Germany, Switzerland, and the UK were involved. The results were published in the renowned journal Science.
Electrons can be in a superposition state in which they interfere with each other quantum mechanically, in effect reinforcing or annihilating each other. Because the dynamics of electron states is extremely fast, the observation of this phenomenon has to take place in the range of attoseconds, an unimaginably short period of time: An attosecond is 10 -18 second, that is, as much shorter than a second as a second is shorter than the whole age of the universe.
To observe such ultrafast processes, the team used a “stopwatch” co-developed by JProf. Helml. They observed the Auger-Meitner effect, a de-excitation process that occurs in all lighter elements and thus also in organic molecules. In the experiment, the research team directed X-rays at the gas nitrogen monoxide. An electron in a shell close to the nucleus is thereby raised to a high-energy state and remains just bound to the atom – there are different variants of this intermediate state, all of which the X-rays can trigger in the molecule at the same time. These are the nuclear excited states of the molecule. "To avoid structural damage, for example breaking apart into its constituents nitrogen and oxygen, the molecule has to ‘calm down’ quickly," explains JProf Helml. To do this, another electron fills the hole that has been created and is drawn closer to the nucleus. This releases additional energy, which is picked up by a third electron and allows it to leave the atom – this electron is called the Auger electron.
Signs of coherent energy state
With this “stopwatch” the physicists were now able to measure how many Auger electrons were emitted at what time and at what angle, and what energy they possessed (see figure). This created an exponential curve in which a large number of electrons are emitted at the beginning and ever fewer with the passage of time.
In this experiment, the research team was able to make this measurement in nitric oxide for the first time. During the analysis, the researchers also made an unusual discovery: At a point in time when the number of electrons emitted should decrease, it actually increased again for a few attoseconds; so the curve showed an intermediate crest. When the photon energy of the X-ray pulse changed, the researchers were able to measure a modulation of this feature, representing an indication of a coherent energy state.

“By tuning the photon energy, we can deliberately intensify or attenuate the coherence. This gives us temporal control of the decay of the excited state,” explains JProf. Helml. "Besides that, the time-resolved energies of the emitted Auger electrons are very sensitive probes for short-lived transition states of the molecule and can reveal a lot about its fundamental properties.”
Collaboration with Stanford University
The measurements were carried out at the SLAC National Accelerator Laboratory in the USA under the leadership of Stanford scientists Siqi Li, Taran Driver, and James P. Cryan. SLAC has one of only five X-ray lasers worldwide that cover the required high-energy range. The researchers' observations could make it possible in the future to selectively produce such superposition states and to examine them more closely. This could, for example, enable researchers to draw conclusions about the exact processes involved in the damage to biological samples, such as proteins or DNA, or could also enable targeted control of binding sites in molecules.
Bio
Since August 2018, Wolfram Helml has been a junior professor in the Department of Physics at TU Dortmund University. He specializes in accelerator and X-ray physics and is based at the synchrotron light source DELTA (Dortmund Electron Accelerator). He comes from Linz on the Danube in Austria. Helml studied physics at the Alma Mater Rudolphina in Vienna, where he graduated with a diploma thesis in theoretical particle physics. For his dissertation, he switched to experimental particle physics at the Max Planck Institute of Quantum Optics in Garching, where he completed his doctorate with distinction in 2012. With a Marie Curie grant from the European Union, Helml then went to the Stanford Linear Accelerator in California, USA, for almost two years and then conducted research at the Technical University of Munich (TUM). Most recently, at LMU Munich, he headed the Laser-driven Undulator X-ray Source sub-project at the Center for Advanced Laser Applications (CALA).
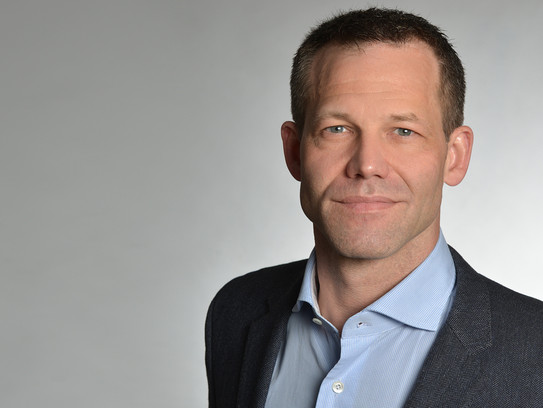